RG-II
.........................................................................................
Introduction
Walls of growing plants are extremely complex and sophisticated composite materials incorporating a dynamic assembly of polysaccharides, proteins and phenolics. Among the polysaccharides, the pectins encompass a group of acidic heteropolysaccharides; they offer a repertoire of structural complexity associated with the occurrence of, at least, three specific domains. Whereas most of these domains are notable for their structural heterogeneity, one of these, the so-called Rhamnogalacturonan-II (RG-II) exhibits a remarkable conservation throughout the plant kingdom. RG-II is thought to be the most complex plant polysaccharide on Earth (MW 5-10 kDa); its occurrence and strong conservation may indicate that it plays a major role in the structure and growth of higher plants
.
Knowledge of the primary structure of RG-II has been largely based on two approaches:
1/ glycosyl linkage composition (determined by methylation analysis) and
2/ identification of various oligosaccharide fragments with mass spectrometry (obtained by mild acid and/or enzymatic hydrolysis of RG-II followed by chromatographic purification).
RG-II has been best described as a homogalacturonan backbone of roughly seven to nine residues bearing four oligosaccharide sidechains, A-D
.
A preliminary molecular mechanics study
of the four oligosaccharide sidechains of RG-II explored the stable orientations of all of the glycosidic linkages of the sidechain sugars as well as the puckering preferences of the apiosyl and aceric acid five-membered rings. The preferred conformers were partially validated by the sequential nuclear Overhauser effects (NOEs) described in the first NMR investigation
of the intact saponified and borohydride-reduced RG-II monomer, mRG-II-ol). This latter study placed the A-sidechain at the penultimate a-GalpA residue from the non-reducing end and revealed some long-range contacts. However, spectral assignments relied heavily on the available primary structure which has been modified since
. Concomitantly, better quality proton-carbon chemical shift correlation data would be acquired to allow more secure assignment of many of the spin systems.
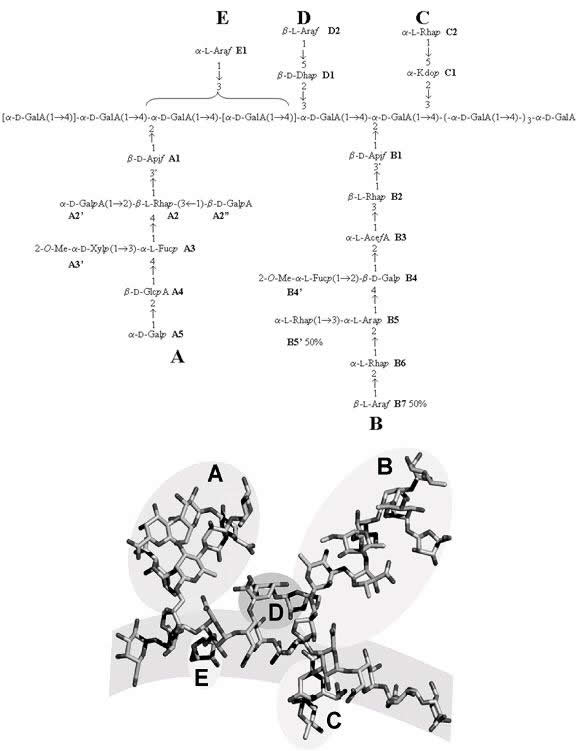
Fig. 1 Schematic representation of the primary structure of RG-II monomer (mRG-II). Since its first identification in 1978, the primary structure has been studied mainly by methylation analysis and mass spectrometry of its oligisaccharides from its partial degradation. Side chains (A-D), as well as the main chain of polygalacturonic acid, have been identified and characterized.
In spite of this, a large body of NMR spectral data has been collected, especially concerning the assigned NOEs, and it has been applied to the preferred conformation of mRG-II in solution. The conformational analysis used the previously reported adiabatic energy surfaces along with the locations of the local energy minima for all disaccharide components of RG-II. These data, along with the NOEs, were combined to determine possible time-average conformations of monomer mRG-II in solution. Two families of conformers, stemming from the two possible diastereospecific assignments of one hydrogen atom were obtained. Both of them are in agreement with other experimental data like the general shape of the molecule and the accessibility of apiose in A-chain. When calculated distances were compared with the NOEs, good agreement was obtained, at least qualitatively in most cases; the intensity of the expected NOEs deviated from the experimental ones only for a very few interactions. Considering on the one hand that the NOEs correspond to a time-averaged or virtual structure, and on the other hand, that the isolated-spin approximation had been applied to systems with nearby protons, we considered the agreement very good. The study has been an iterative process between the computational study and the NMR assignments. Thus, initial conformations for RG-II or its fragments led to the revision of known signals and the assignment of new ones that, in turns, resulted in a new set of NOEs constraints or the modification of the previous ones. The resulting structures gave slightly longer distances after minimisation than expected in the case of most-long range NOEs. Nevertheless, the optimised conformers could be considered as good starting geometries for further studies on the molecular dynamics of the RG-II monomer and the conformational analysis of the RG-II dimer
.
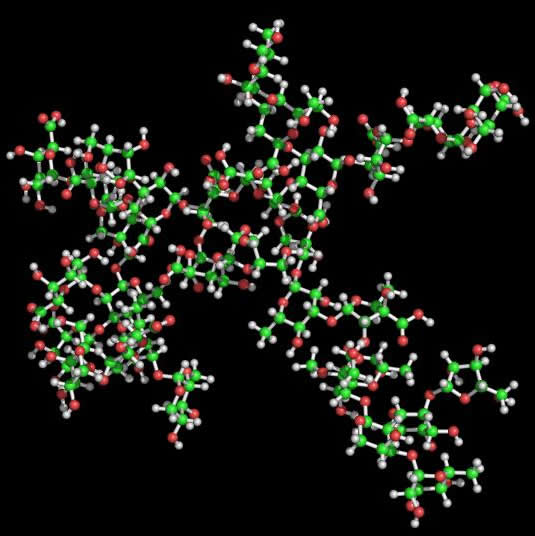
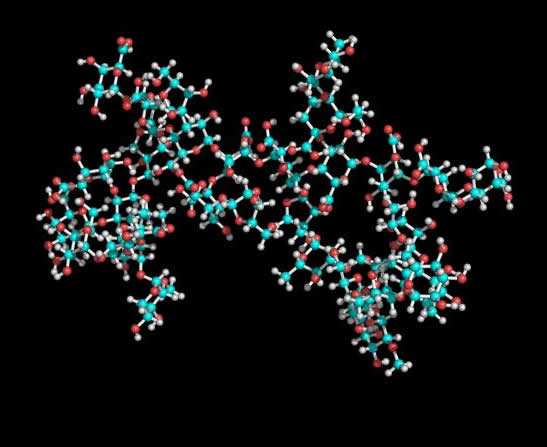
Fig. 2 Representation of the two lowest energy conformers of RG-II
3D representation of the two lowest energy conformations of the RG-II monomer found are given. The general shape of the mRG-II resulted in a quite flat structure, with a length of about 37 Å for the main chain (horizontal diameter) and roughly the same length between the longest side chains (A and B) (vertical diameter). The general shape was a disk, with a thickness of about 17 Å, what is in agreement with the results obtained by translational self-diffusion and light-scattering. Preliminary mRG-II molecular dynamics results confirmed the stability of the starting geometries. The main chain maintains a stiff shape close to an extended helix, with a mean extension of around 38 Å. This value is close to the expected one for a 9-unit pectate helix adopting a 2-fold (38.2 Å) or 3-fold (39.9 Å) conformation. Moreover, galacturonic glycosidic torsion angles explore the minima found in molecular mechanics. According to these results, the presence of RG-II side chains does not affect the extension and direction of HGA. This fact suggests that the interaction of galacturonic acid with calcium cations in muro could initiate the approaching of mRG-II as previous step to the dimerisation with boron. Thus, two molecules of mRG-II cross-linked by a boron atom (Figure 6) will serve as good starting geometries for further refinement using molecular dynamics, once NOE constraints could be applied.
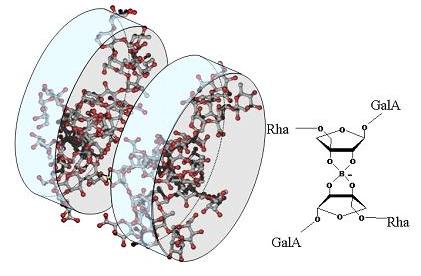
Fig.3 Tentative representation of the 3D organizsation of RG-II dimer (dRG-II). RG-II is present in plant primary cell walls predominantly as a dimmer in which two apioses (belonging to the A-chain) are cross-linked by a 1:2 borate-diol ester. Two diastereoisomers are possible, depending on the orientation of monomers (main chains arranged in parallel or anti-parallel fashion). According to the proposed 3D structure form mRG-II, apiose in A-chain is not located on the centre of the molecule. Thus the anti-parallel complexation would lead to an extended structure, whereas the parallel one would maintain the surface of the monomer, which is in agreement with Atomic Force Microscopy results and hydrodynamic features of the dimer
The structure-function relationship of the RG-II fragment, is just emerging from the knowledge of its three-dimensional structure, both in its monomeric and dimeric form
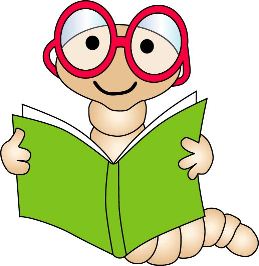
. The significant structural feature is certainly its “
disk-like” shape that results from the most complex three-dimensional arrangement of 31 monosaccharides units, arranged in five side chains of unequal importance, flanking a typical pectic backbone. As with any other complex oligosaccharides, there is room for some conformational flexibility about each glycosidic linkages. Overall, a well defined tertiary structure seems to emerge for mRG-II as well as for dRG-II in which boron complexation accompanied by Ca
2+ pairing provide further stabilisation of the two disks. This results in an architectural motif that has never been depicted for any of the plant-cell components. This motif must be considered as a “
clef de voute” that provides stable links in key regions within the pectic network. This is in contrast to pectic backbone for which its contribution to network architecture is mediated throughout the action of enzymes and is therefore time dependent. Once bio-synthesised and located in the cell wall, RG-II, with its insensibility towards all cell wall enzymes, offers a structure (mRG-I) that will remain stable for all the life time of the cell-wall. When this monomeric structure (presumably linked to the pectic backbone) interacts with another same structure throughout Boron and Ca
2+ binding, a very stable
spatial anchor is formed that will resist to any
temporal changes
. This is of course in contrast to the dynamical interactions that are undergone by the HGA moiety, where parallel and anti-parallel chain pairings may occur to stabilize in a highly time dependence fashion the gel constituent of an expanding medium. The observation that any alteration in the structure and/or binding abilities of RG-II results in severe growth defects that relates to mechanical instabilities is obviously in line with the essential architectural role that RG-II may have in the cell wall.